Light Emitting Diode (LED)
Light emitting diodes are some of the most common components in electrical circuits and electronic systems. A light emitting diode, commonly referred to as an LED, is a type of diode that emits light as electric current passes through it.
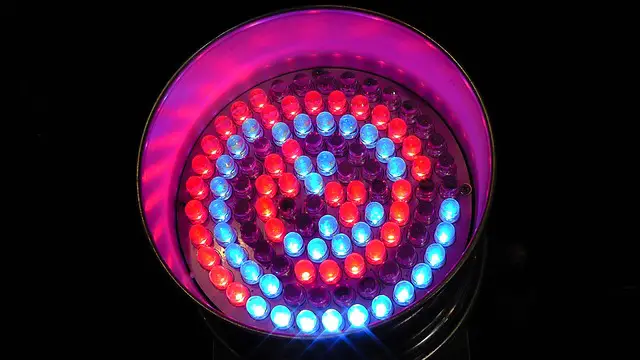
What Are Light Emitting Diodes?
Like most diodes, LEDs are semiconductor devices that are based on the principle of P-N Junctions. LEDs are one of the most common types of diodes due to their usefulness and efficiency.
LEDs produce a narrow band of visible or infrared light, and are extremely efficient at converting electrical energy into light or infrared radiation.
Like other kinds of diodes, LEDs will only allow current to pass when they are forward-biased. This means that they prevent current in the opposite direction, and therefore have polarity. The primary function of most LEDs is to produce light, but like other diodes, they also rectify current. If an LED isn’t working, check to make sure that it’s not being reverse-biased. You can check the polarity of a diode by looking for the flat spot on the base, located on the side of the negative terminal (cathode).
LED Symbol
The electric schematic symbol for an LED is based on that of a diode. It is distinguished from the symbol for a regular diode by the presence of two arrows indicating the emission of light.
LED Efficiency and Usage
LEDs are amazing because the light they produce is of a narrow band. As a comparison, incandescent bulbs produce light along a large spectrum, including lots of infrared radiation, which generates heat when absorbed. This is why light bulbs can be dangerously hot, and also what makes them so inefficient as light producers.
In contrast, instead of producing heat and light of many different frequencies like an incandescent bulb, LEDs produce only light (no heat), which is of a specific color. This makes them much more efficient, cooler, and even safer than incandescent bulbs for most purposes.
Since they are made of pure solid state semiconductor materials, their lifecycles are extremely long. An LED will outlast virtually every other type of commercial lighting option.
Due to their efficiency, LED bulbs are replacing incandescent and fluorescent bulbs in household and commercial applications. Greater efficiency and a longer lifecycle means lower energy costs, lower maintenance costs, lower carbon footprint, reduced emissions, and increased stability of the energy grid.
Because the light produced by an LED is of a specific color, they can also be customized for further benefit to specific applications. LED grow lights used in greenhouses are particularly effective because they can produce light that specifically targets the absorption patterns of chlorophyll, and even optimized for a specific plant species or strain.
LED Construction
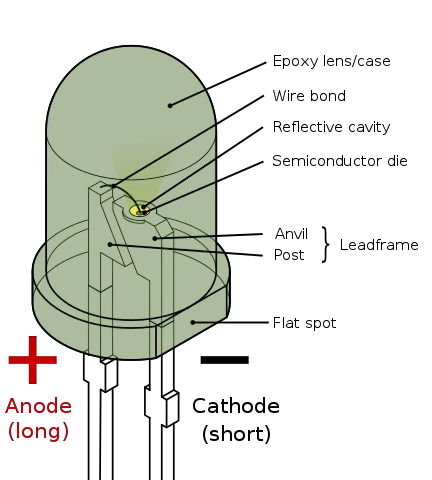
LEDs are designed to emit light, but also to be rugged enough to function on the outside of devices. The actual semiconductor junction must be protected, and is therefore contained within an epoxy case that allows light through while protecting the diode junction itself. The case may also be colored to enhance the color produced by the LED.
The positive terminal (anode) typically has a longer lead, and the negative terminal (cathode) is usually marked by a flat area for quick reference.
Inside the case, the semiconductor die is the site of the actual semiconductor P-N Junction.
Behind the die is a reflective cavity that reflects light so that more light reaches the outer case. Without the reflective cavity, much of the light would be directed at angles that would not contribute to the light escaping the case.
The lead frame is a metal structure that provides the electrical connection and mechanical support to the die and reflective cavity.
The lead frame has two parts; an anvil that is (usually) connected to the cathode and a post that is (usually) connected to the anode. In some designs, this orientation is reversed, so a best practice is to always rely on the flat spot to determine the polarity, rather than the location of the anvil. The anvil is larger than the post and holds the reflective cavity, providing mechanical support.
The wire bond is the connection between each side of the semiconductor die and the lead frame.
How Do LEDs Work?
LEDs, like most diodes, are essentially P-N junctions at heart.
P-N junctions are devices that are made by doping a semiconductor material.
One side of the junction contains p-type dopants, which are essentially atoms that have one less valence electron than the bulk semiconductor material. For example, silicon has 4 valence electrons so a silicon-based p-type material is doped with atoms that have 3 valence electrons, like boron.
The other side of the junction contains n-type dopants, which are atoms that have one more valence electron than the bulk semiconductor material. Phosphorous has 5 valence electrons, one more than silicon, and is one of most common n-type dopants in silicon based devices.
The actual ‘junction’ can refer to the entire device, but more specifically refers to the junction ‘point’, or cross-section, between the p-side and the n-side.
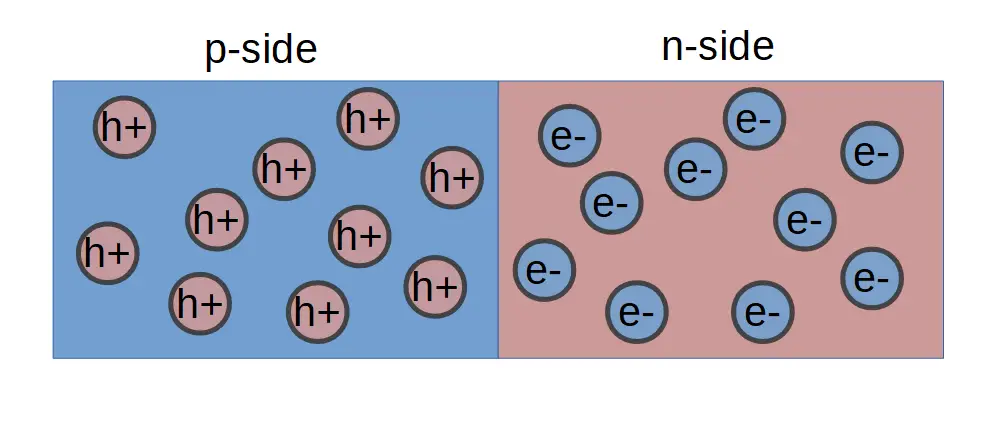
In the lesson on P-N junctions, we learned that current can only travel through the diode in one direction. Current flows when a forward bias is applied, which means that the positive terminal of the voltage or power source is on the p-doped side, and the negative terminal is on the n-doped side.
When a forward bias is applied in a diode, current flows in a slightly different way than it does in an ordinary wire. Instead of electrons simply moving from one side of the diode to another, electrons recombine with holes at the center of the junction. This is called carrier recombination.
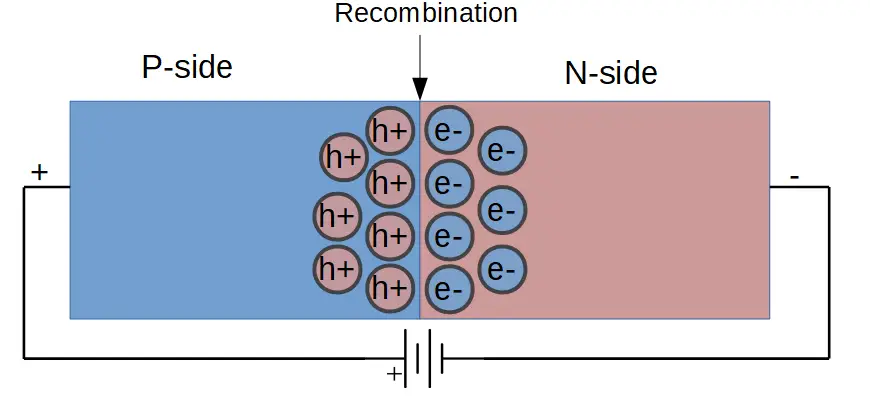
Holes (which are positively charged) are pushed toward the center of the junction by the positive terminal of the voltage source. Electrons (which are negatively charged) are pushed toward the center of the junction by the negative terminal of the voltage source.
As electrons and holes meet at the center of the junction, they combine in a process called carrier recombination.
Carrier Recombination in LEDs
When electrons and holes meet in the middle of the junction, the electrons are at a high energy level, which corresponds to the conduction band of the semiconductor. Holes are at a lower energy level, corresponding with the valence band of the semiconductor.
In order to recombine, the electron must lose some energy and occupy the hole. It does so by emitting a photon, which is a particle of electromagnetic radiation in the form of heat or light.
All diodes function in a similar way; by emitting energy during carrier recombination at the junction.
The unique characteristic of an LED is that the junction is specifically engineered so that it only produces light, in either the visible or infrared band. In other words, the frequency of the photon is within the narrow band on the full spectrum of electromagnetic radiation that corresponds with either visible or infrared light.
Forward Voltage of a Light Emitting Diode (LED)
Like other diodes, light emitting diodes have a minimum forward voltage required for the LED to pass current. This forward voltage is about 1.2 Volts for the lowest energy diodes (infrared), but increases to around 4 volts for higher energy diodes (blue and white).
If the forward voltage is not met, current will not pass through the diode, and the circuit will function like an open circuit.
When a forward bias is applied that is greater than the forward voltage, current will begin to flow through the diode. The diode will cause a voltage drop that is equal to the forward voltage. Like resistors, a greater current will result in a larger voltage drop. If the diode’s voltage drop is a significant factor in circuit design, it is important to use that LED’s I-V curve to determine the voltage drop.
LED Colors
Up to this point, we’ve focused on silicon based doping to produce P-N junctions. However, a simple doped silicon crystal is not capable of producing a diode that emits visible light with good efficiency. Instead, crystals of more complex semiconductors are used to manufacture LEDs that produce visible light.
Most LEDs are gallium based, and are known as III-V semiconductors because they combine elements from Group III and Group V from the periodic table of elements.
Group III | Group V |
Boron (B) | Nitrogen (N) |
Aluminum (Al) | Phosphorous (P) |
Gallium (Ga) | Arsenic (As) |
Indium (In) | Antimony (Sb) |
LED Color | Semiconductor | Wavelength | Forward Voltage |
Infrared | GaAs | 850-940nm | 1.2v |
Red | GaAsP | 630-660nm | 1.4v |
Amber | GaAsP | 605-620nm | 2.0v |
Yellow | GaAsP:N | 585-595nm | 2.2v |
Green | AlGaP | 550-570nm | 3.5v |
Blue | SiC | 430-505nm | 3.6v |
White | GaInN | 450nm | 4.0v |
The exotic chemical compositions of LEDs are used in order to engineer the LED’s band structure. The band structure determines the energy of the photons emitted during electron-hole recombination. This energy then determines the frequency and wavelength, and therefore, the color of light produced by the LED.
Energy, Light, and Color
(Note: This section presents an introduction to the relationship between the energy of a photon and the color of light produced. If you’re familiar (or uninterested), feel free to skip ahead).
The frequency of light is determined by the energy band gap between the conduction and the valence band. The greater the gap, the higher the energy of the photon produced during electron-hole recombination. The frequency of the light is determined by a mathematical relation called Planck’s equation:
E=hf
Where E is the energy of the photon of light, f is its’ frequency, and h is a constant (Planck’s constant) that is actually defined by this relation (i.e. Planck’s constant is the ratio of the energy of a photon to its’ frequency).
The following figure shows the relation between the frequency, wavelength, and location on the electromagnetic spectrum:
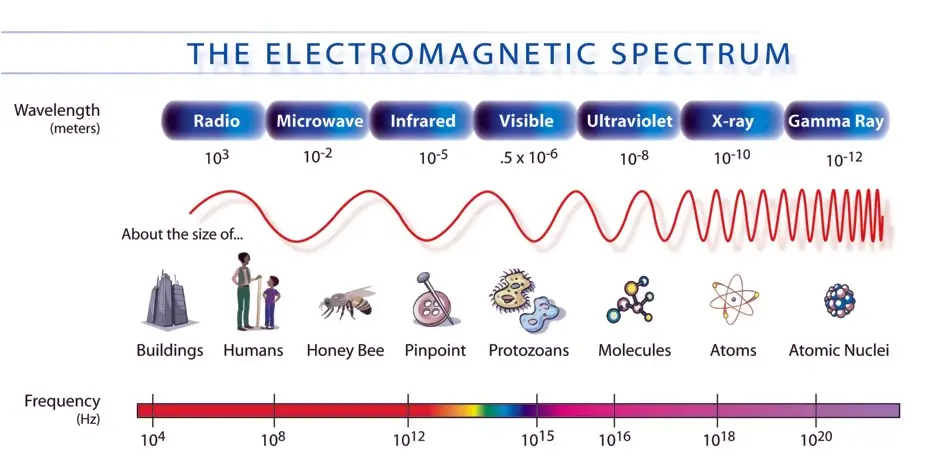
The frequency and wavelength of light are related to each other by the speed of light:
\lambda = \frac{c}{f}
You can also see that visible light occupies only a small band of the electromagnetic spectrum:

So an LED needs to product photons that fall within the visible spectrum. It does this by having an energy band gap corresponding with the energy of the photons:
E = hf = \frac{hc}{\lambda}